Biportal Endoscopic Transforaminal Interbody Fusion for Lumbar Adjacent Segment Disease: An Illustrative Case and Literature Review
Article information
Abstract
Posterior lumbar fusion surgery is a well-established procedure for treating degenerative lumbar spine diseases. Despite its surgical success over the decades, adjacent segment degeneration persisted as a problem, causing significant morbidity in patients. Various surgical fusion techniques, including open and minimally invasive procedures, have been reported for treating adjacent segment disease. Recently, endoscopic lumbar interbody fusion, including fully endoscopic lumbar interbody fusion through the uniportal approach and biportal endoscopic transforaminal lumbar interbody fusion (BE-TLIF), has been attempted as a minimally invasive surgical technique for lumbar fusion. This study describes the BE-TLIF technique and presents a review of the literature on this technique for treating adjacent segment disease. Our case illustration demonstrates that BE-TLIF could be a viable minimally invasive technique for addressing adjacent segment disease in revision lumbar spinal fusion surgery.
INTRODUCTION
Posterior lumbar fusion surgery, a cornerstone treatment for degenerative lumbar spine pathologies, has achieved sustained success over the years. However, it is not without complications. Notably, adjacent segment degeneration (ASDeg) can manifest postoperatively, contributing to significant patient morbidity [1-3]. ASDeg is characterized by the emergence of degenerative changes at vertebral levels adjacent to those previously operated on [4], manifesting symptoms necessitating further surgical intervention—termed adjacent segment disease (ASDis) [5].
Research in biomechanics and clinical studies has identified spinal fusion as a potential aggravator of intradiscal pressure in adjacent segments, precipitating disc degeneration and other degenerative alterations [6-8]. Common pathologies observed adjacent to fused segments include listhesis, instability, hypertrophic facet joint arthritis, herniated nucleus pulposus, and stenosis [9]. The reported incidence of ASDeg following arthrodesis varies widely, with a meta-analytic incidence rate of 5.9% per year for ASDeg and 1.8% per year for ASDis in a pooled analysis of 1,551 patients [5].
Risk factors for adjacent segment pathology extend from patient-specific characteristics—such as age, pre-existing degeneration, and surgical history—to technical aspects of the fusion procedure, including the number of fused segments and the surgical technique employed [5,10,11]. Other risk factors for adjacent segment pathology such as osteoporosis, body mass index, smoking, activity level, and alcohol consumption may have been suggested [5]. Long fusions also increase the vector force, imparting more stress on the non-fused segments. Notably, biomechanical stress distribution, as shown by Chen et al. [11] through finite element modeling, is exacerbated by the length of fusion, with longer constructs transferring increased stress to adjacent segments [3,11-13]. Additionally, the alignment of the fused segments has been implicated in the biomechanical stress on adjacent segments, influencing the rate of degeneration [2,3]. A prospective, randomized controlled trial highlighted that 38% of patients undergoing fusion surgery developed ASDeg, a stark contrast to the nonsurgical control group, suggesting that surgical intervention may accelerate the degenerative process beyond natural progression [13].
Surgical techniques have evolved, incorporating both traditional and minimally invasive approaches, with recent developments in endoscopic lumbar interbody fusion, such as full-endoscopic lumbar interbody fusion and biportal endoscopic transforaminal lumbar interbody fusion (BE-TLIF), offering promising outcomes [14-16]. These techniques have been associated with better functional recovery and reduced recovery times when compared to traditional open fusion methods [17,18]. More recently, endoscopic spine surgery either uniportal or biportal techniques has been used to treat ASDis [16,19,20].
This article presents a detailed case study of adjacent segment pathology following prior open lumbar fusion surgery, wherein BE-TLIF was utilized for the subsequent lumbar interbody fusion procedure, illustrating both the condition and the surgical response.
CASE REPORT
A case of a 57-year-old woman with persistent back pain and bilateral radiculopathy, exacerbated in the right leg, is presented. The patient had a history of L4–5 spondylolisthesis, for which she underwent open posterior lumbar interbody fusion a decade prior. Recent magnetic resonance imaging (MRI) scans revealed degenerative spondylolisthesis with severe central stenosis at the L3–4 level (Figure 1A and B). Temporary relief was achieved through transforaminal epidural steroid injection, but symptoms recurred. We performed a BE-TLIF at L3–4, and revision of previous instrumentation. through Wiltse approach using 2 paramedian skin incisions and percutaneous pedicle screws were inserted at L3. The postoperative MRI showed adequate neural decompression (Figure 1C and D) and postoperative plain radiography revealed that spondylolisthesis was reduced well (Figure 1E-H). Postoperatively, the patient’s symptoms were significantly improved.
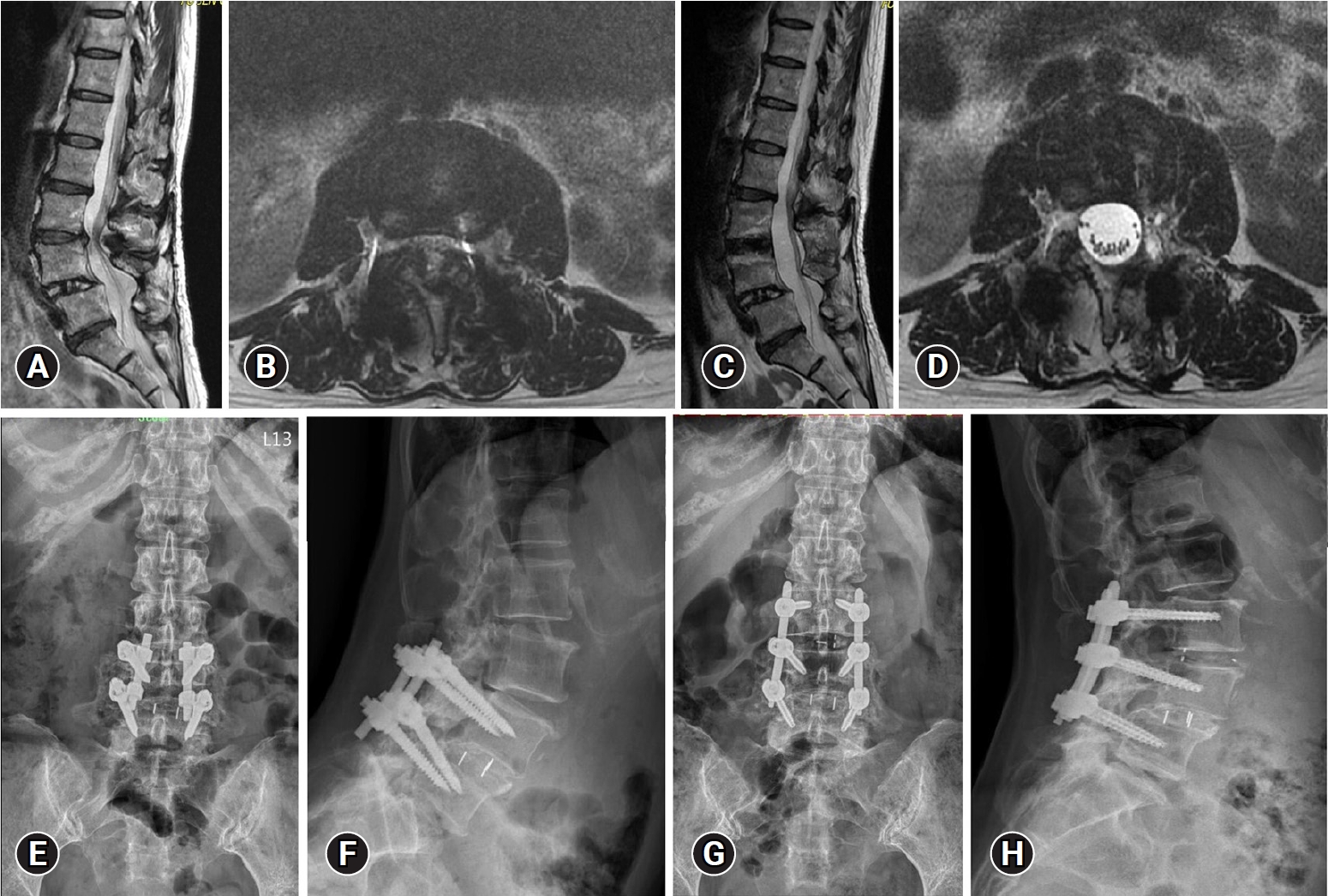
Radiologic images of a 57-year-old female patient. (A) Preoperative magnetic resonance imaging shows degenerative spondylolisthesis with central herniation of the intervertebral disc at L3–4. (B) After biportal endoscopic transforaminal lumbar interbody fusion, the spondylolisthesis was well reduced. Severe central stenosis at L3–4 (C) was decompressed after surgery (D). (E, F) A preoperative plain radiograph shows previous fusion at L4–5 with spondylolisthesis of L3–4. (G, H) Eighteen months after surgery, the construct and alignment were well-maintained.
The informed consent was obtained from the patient prior to publication.
1. Surgical Method and Instrumentation
The surgical procedure entailed a biportal endoscopy system, necessitating a camera, irrigation system, and specialized tool kits. Essential components included a waterproof surgical drape, a radiofrequency ablation console, and probes for tissue dissection, cauterization, and hemostasis. General spinal surgical instruments were used, including high-speed drills, chisels, and curettes, facilitating laminectomy, facetectomy, and endplate preparation. The procedure also involved percutaneous pedicle screw insertion under C-arm fluoroscopic guidance.
2. Anesthesia and Position
General endotracheal anesthesia was preferred for optimal blood pressure control. The patient was positioned prone on a radiolucent Jackson table to enhance radiographic visualization.
3. Surgical Procedures
First, we made 2 longitudinal skin incisions, paramedian, above the previous instrumentation level (L4–5). Using Wiltse intermuscular approach, the old pedicle screws at L4 and L5 were removed. Removing the old pedicle screws first can reduce the interference from existing implants during endoscopic procedures, thus enhancing maneuverability. The operational space formed by water pressure during surgery did not cause interference. While the water flow may increase, under good pressure control from the pump, both the clarity of the surgical field and the surgical procedure remain unaffected. A 5-mm-long skin incision for an endoscopic portal was made close to the left L3 pedicle level of the medial pedicular line and the wound for screw removal was used on the working portal over the level of L4 pedicle. These skin incisions were also used for ipsilateral pedicle screw insertion (Figure 2). Dilators were inserted through the working portals and the lower portion of the cranial lamina (L3) was gently dissected using a radiofrequency probe to create an endoscopic base camp. Ipsilateral unilateral laminotomy of the upper and lower lamina with an ipsilateral facetectomy was performed until full exposure of the ligamentum flavum (LF) from the proximal end to the distal end.
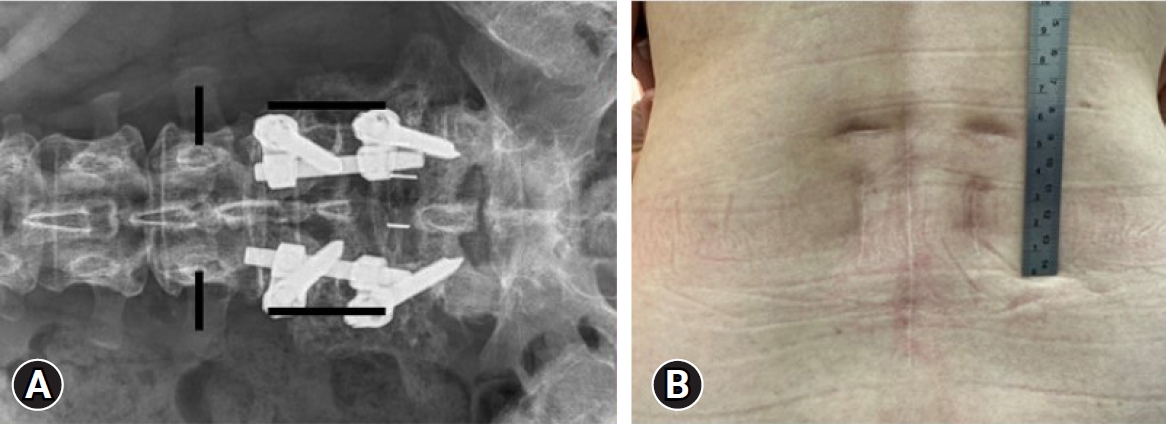
(A) A skin incision designed for biportal endoscopic transforaminal lumbar interbody fusion to treat adjacent segment disease at L3–4. (B) A clinical photograph of the patient shows a midline longitudinal wound scar from primary open posterior lumbar interbody fusion accompanied by 4 separate wounds for biportal endoscopic transforaminal lumbar interbody fusion.
We performed en bloc removal of ipsilateral LF, identifying the axillary area of left L3 root, lateral border of dura and left side L4 traversing root. The unilateral inferior articular process was removed using Kerrison punches and osteotomes. The superior articular process was partially removed. Bone chips from lamina and facet were collected for fusion materials. Then we performed “over-the-top” decompression starting from the spino-laminar junction of L3, followed by sublaminar bone drilling at contralateral (right) side of L3 lamina and upper border of L4 lamina. Contralateral LF was removed piece by piece using disc rongeurs and Kerrison punches.
We then identified the axillary area of right side L3 exiting root, lateral border of dura and traversing root (L4) (Figure 3A). We gently medially retracted ipsilateral dura and identify the L3–4 disc space (Figure 3B). Annulotomy was done using a blunt knife and an radiofrequency (RF) ablation probe. The disc materials were removed using pituitary forceps and shavers. We performed complete endplate preparation under the endoscopic view. The endoscope could be inserted into disc space. The dissection plane between the cartilaginous endplate and osseous endplate is explored under a clear, magnified endoscopic view (Figure 3C). The cartilaginous endplate is separated from the osseous endplate using angled curettes and chisels. After confirmation of complete endplate preparation, we applied one K-wire lateral to the border of dura and another K-wire distal to left L3 exiting root to create a space for cage insertion. Under endoscopic view, allogenous bone chips and artificial bone substitute were inserted. Continuous saline irrigation should be stopped during the insertion of fusion materials. After serial size trials, a 10*10*30 mm PEEK cage (ReBorn Essence Inc., New Taipei City, Taiwan) was inserted through the working portal (Figure 3D). We removed K-wires and checked the position of the cage under C-arm fluoroscopy.
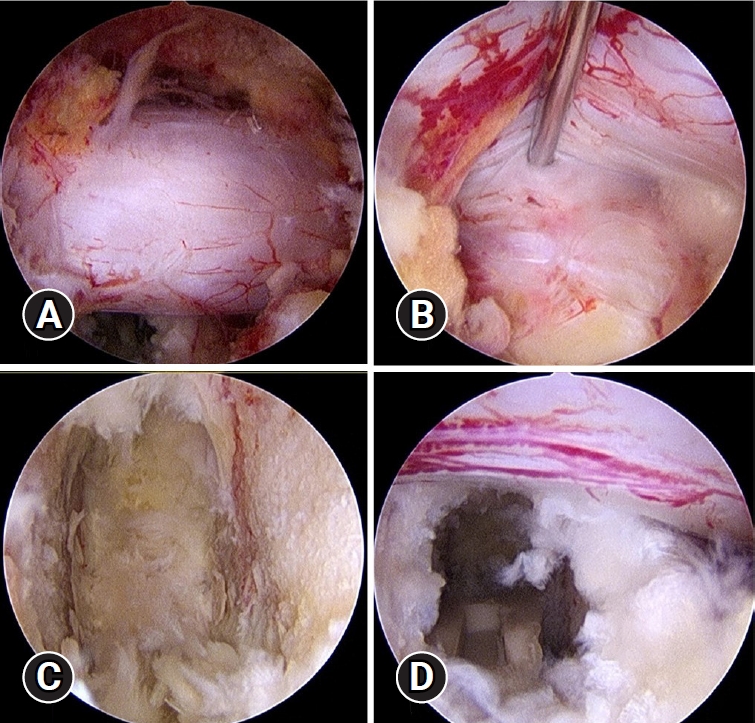
Endoscopic images during neural decompression and interbody fusion procedure. (A) Over-the-top view after bilateral neural decompression, in which we then identified bilateral lateral borders of the dura sac. (B) The ipsilateral dura and L4 nerve root were retracted, and we identified the L3–4 disc space. (C) Final view of the endoscopic endplate preparation. (D) A polyetheretherketone cage was inserted under endoscopy.
Finally, we marked the lateral border of right side L3 pedicle and made a 1.5-cm wound for L3 pedicle screw. After percutaneous application of all 6 screws at L3, L4 and L5, the rods were inserted bilaterally and the nods were fastened. A drainage catheter was inserted to prevent postoperative epidural hematoma
DISCUSSION
ASDis presents significant challenges in post-lumbar fusion surgery care. Traditional open spinal surgeries, while effective, often lead to complications such as paraspinal muscle damage, muscular denervation, epidural fibrosis, and increased hospital stays and costs [21-25]. This has led to the development of minimally invasive spine surgery (MISS) techniques to mitigate these issues [26,27]. Minimally invasive tissue-sparing approaches become the standard practice and a preferred technique in spine surgeries. The evolution of MISS over the past 2 decades has shifted from air-based to water-based mediums, with the latter offering improved bleeding control and clearer imaging [27,28]. The better mobility of the endoscope and instrument creates a wider working space and a better evaluation of neural decompression and fusion bed preparation. Unilateral endoscopic lumbar interbody fusion (ULIF) takes advantage of endoscopic system which preserves the paravertebral muscle and the bony structures as much as possible. This technique combines endoscope and the standard working spine instrument such as the Kerrison punch, reamers, nerve retractors, etc. ULIF uses the biportal endoscopic technique which delivers higher mobility, bigger working space and direct vision for cage insertion which provides surgeons a less invasive way for fusion than open surgery [29].
BE-TLIF procedure, in particular, demonstrates advantages in reducing surgical trauma, hospitalization time, and blood loss, while improving patient outcomes [14,30-34]. The visual analogue scale scores for back and leg pain and Oswestry Disability Index scores were significantly improved after BE-TLIF [32]. This method utilizes smaller incisions, leading to less postoperative trauma and quicker recovery [30]. The use of a bipolar RF thermos-controlled ablator instead of traditional electrocautery minimizes thermal injury to paravertebral muscles and neural elements, potentially reducing surgical-site infections and enhancing vascular cauterization. This is a potential way in reducing surgical-site infection, as it does not have surgical smoke and diminishes wound contamination. Additionally, efficient vascular cauterization without nerve injury can be obtained in cases of bleeding in the microvasculature around the dural sac [30-32].
The dual surgical ports in BE-TLIF allow dynamic instrument handling, optimized field vision, and continuous fluid irrigation, facilitating safe and efficient neural decompression and endplate preparation [17]. To increase the contact surface between the graft and the vertebral body and the chance of successful spinal fusion, a substantial proportion of disc materials should be removed without any vertebral endplate damage. Thus, the biportal endoscopic technique can provide a clean and magnified real-time surgical visualization, allowing for complete visual inspection of all the fusion beds. Continuous fluid irrigation may prevent the accumulation of heat energy that can cause thermal necrosis of the vertebral bone and end- plate, induced by using a power drill and electrocautery. For endoscopic lumbar interbody fusion, although there is still no clear evidence, complete visual inspection of endplate trabecular bone, dispersion of thermal energy by continuous fluid irrigation, and endplate preparation using RF ablation may potentially increase the chances of successful interbody spinal fusion. Concerns regarding the washout of graft materials due to continuous fluid irrigation have been addressed, with studies indicating an acceptable spinal fusion rate over 80% at 12 months postoperatively which confirmed the non-inferiority of BE-TLIF compared to minimal invasive transforaminal lumbar interbody fusion (MIS-TLIF) [35,36].
Disadvantages of the BE-TLIF technique are that it may be more difficult compared to conventional open surgery or MIS-TLIF, and the learning curve for endoscopic spine surgery is steep [14-19,37-40]. Several studies focusing on complications and poor prognosis after conventional posterior lumbar instrumented fusion surgery reported that longer operation time, greater blood loss, more extensive surgical levels (≥3 segments), and longer hospitalization duration were independent risk factors [37-41]. While BE-TLIF has a steeper learning curve and longer operation times compared to conventional surgeries [42], it offers lower estimated blood loss and reduced need for perioperative transfusions, counterbalancing these challenges [18,37]. These findings indicate that longer operation time, a risk factor for postoperative adverse events and poor prognosis, may not apply to BE-TLIF [41,43].
CONCLUSION
The presented case illustrates the efficacy of BE-TLIF as a minimally invasive approach for managing ASDis in revision lumbar spinal fusion surgeries. This technique not only provides direct decompression of neural elements and stabilization but also allows direct viewing of cage insertion and fusion, thus presenting a comprehensive solution for patients with ASDis. The continual evolution and refinement of these techniques underscore the potential for improved patient outcomes in spinal surgery.
Notes
Conflict of Interest
The authors have nothing to disclose.
Funding/Support
This study received no specific grant from any funding agency in the public, commercial, or not-for-profit sectors.